Cardiovascular Pharmacology
Cardiovascular pharmacology focuses on the fundamental mechanisms of cardiovascular cells and how drugs influence the heart and vascular system. Researchers in the department exploit novel technologies such as induced pluripotent stem cells, automated electrophysiology and genomics to investigate the causes of sudden cardiac death, congenital arrhythmias and cardiac toxicity of chemotherapeutic drugs.
Our Work
Pharmacogenomics in African-Americans and Other Understudied Populations
Minoli Perera, PharmD, PhD, associate professor of Pharmacology, studies African American pharmacogenomics, which is the study of an individual’s genome to predict how they will respond to drugs.
Investigating the Pharmacogenomics of Chemotherapy Toxicity
Paul Burridge, PhD, associate professor of Pharmacology, studies how a patient’s genetics cause negative responses such as cardiotoxicity to chemotherapy agents.
Cardiovascular Pharmacology Research Labs
Paul Burridge LabInvestigating the application of human induced pluripotent stem cells to study the pharmacogenomics of chemotherapy off-target toxicity and efficacy
Investigating the application of human induced pluripotent stem cells to study the pharmacogenomics of chemotherapy off-target toxicity and efficacy
Research Description
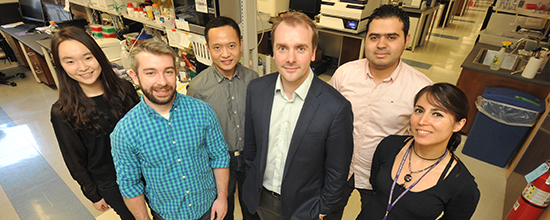
The Burridge Lab studies the role of the genome in influencing drug responses, known as pharmacogenomics or personalized medicine. Our major model is human induced pluripotent stem cells (hiPSC), generated from patient's blood or skin. We use a combination of next generation sequencing, automation and robotics, high-throughput drug screening, high-content imaging, tissue engineering, electrophysiological and physiological testing to better understand the mechanisms of drug response and action.
Our major effort has been related to patient-specific responses to chemotherapy agents. We ask the question: what is the genetic reason why some patients have a minimal side effects to their cancer treatment, whilst others have encounter highly detrimental side-effects? These side-effects can include cardiomyopathy (heart failure or arrhythmias), peripheral neuropathy or hepatotoxicity (liver failure). It is our aim to add to risk-based screening by functionally validating genetic changes that predispose a patient to a specific drug response.
Recent Findings
- Human induced pluripotent stem cells predict breast cancer patients’ predilection to doxorubicin-induced cardiotoxicity
- Chemically defined generation of human cardiomyocytes
Current Projects
- Modeling the role of the genome in doxorubicin-induced cardiotoxicity using hiPSC
- Investigating the pharmacogenomics of tyrosine kinase inhibitor cardiotoxicity
- hiPSC reprogramming, culture and differentiation techniques
- High-throughput and high-content methodologies in hiPSC-based screening
For lab information and more, see Dr. Burridge’s faculty profile and lab website.
Publications
See Dr. Burridge's publications on PubMed.
Contact
Contact Dr. Burridge at 312-503-4895.
Al George LabInvestigating the structure, function, pharmacology and molecular genetics of ion channels and channelopathies
Investigating the structure, function, pharmacology and molecular genetics of ion channels and channelopathies
Research Description
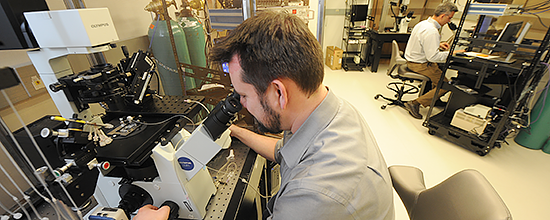
Ion channels are ubiquitous membrane proteins that serve a variety of important physiological functions, provide targets for many types of pharmacological agents and are encoded by genes that can be the basis for inherited diseases affecting the heart, skeletal muscle and nervous system.
Dr. George's research program is focused on the structure, function, pharmacology and molecular genetics of ion channels. He is an internationally recognized leader in the field of channelopathies based on his important discoveries on inherited muscle disorders (periodic paralysis, myotonia), inherited cardiac arrhythmias (congenital long-QT syndrome) and genetic epilepsies. Dr. George’s laboratory was first to determine the functional consequences of a human cardiac sodium channel mutation associated with an inherited cardiac arrhythmia. His group has elucidated the functional and molecular consequences of several brain sodium channel mutations that cause various familial epilepsies and an inherited form of migraine. These finding have motivated pharmacological studies designed to find compounds that suppress aberrant functional behaviors caused by mutations.
Recent Findings
- Discovery of novel, de novo mutations in human calmodulin genes responsible for early onset, life threatening cardiac arrhythmias in infants and elucidation of the biochemical and physiological consequences of the mutations.
- Demonstration that a novel sodium channel blocker capable of preferential inhibition of persistent sodium current has potent antiepileptic effects.
- Elucidation of the biophysical mechanism responsible for G-protein activation of a human voltage-gated sodium channel (NaV1.9) involved in pain perception.
Current Projects
- Investigating the functional and physiological consequences of human voltage-gated sodium channel mutations responsible for either congenital cardiac arrhythmias or epilepsy.
- Evaluating the efficacy and pharmacology of novel sodium channel blockers in mouse models of human genetic epilepsies.
- Implementing high throughput technologies for studying genetic variability in drug metabolism.
- Implementing automated electrophysiology as a screening platform for ion channels.
For lab information and more, see Dr. George’s faculty profile.
Publications
See Dr. George's publications on PubMed.
Contact
Contact Dr. George at 312-503-4892.
Minoli Perera LabPharmacogenomics research in minority patient populations
Pharmacogenomics research in minority patient populations
Research Description
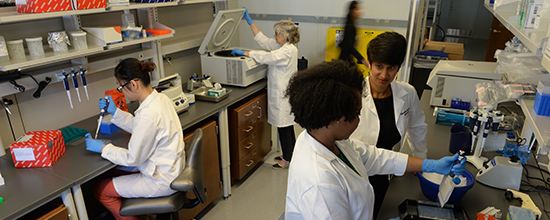
The Perera laboratory focuses on pharmacogenomics (using a patient's genome to predict drug response) in minority populations. Working in this translation research space requires both clinical expertise as well as the use of high-throughput basic science approaches. Our goal is to bring the benefits of precision medicine to all US populations.
The Perera lab has recruited patient populations from around the world. The data collection includes genomic (DNA), transcriptomic (mRNA), pharmacokinetic and clinical data. We then integrate these different data sources to understand genetic drivers of drug response (e.g. genetic predictors of adverse events) as well as disease. By studying minority populations the lab has discovered genetic risk variants that may benefit the implementation of precision medicine in African Americans and others.
Recent Findings
- Warfarin Bleeding Risk Association study: We recently discovered a genetic variant that predispose African Americans to bleeding complications while on anticoagulant drugs. These bleeds occurred even when the patient was within the therapeutic window for the medication. We hope that this new data will help to identify high risk individuals prior to therapy.
- Novel African-specific genetic polymorphisms predict the risk of venous thromboembolism: We discovered a new genetic variant associated with a 2.5 fold increase in risk of developing a blood clot. We went on to show that this SNP significantly affects the expression of a key protein in the coagulation cascade. View article on PubMed.
- Common genetic variant is predictive of warfarin metabolism and gene expression in African Americans: We tested the association of a SNP, previously shown to effect gene expression CYP2C9, for association with warfarin drug clearance (pharmacokinetics). This SNP increased the expression of CYP2C9 (enzyme that metabolized warfarin), hence causing fast clearance of the drug. This African American-specific SNP may help to explain the higher warfarin dose required by African Americans in general. View article on PubMed.
Current Projects
- Genomics of Drug Metabolism: We are using African America primary hepatocytes to understand the genetic regulation of drug metabolizing enzymes that are involved in a majority of drug used in the US.
- Anticoagulant Pharmacogenomics: We are conducting several genetic association studies to understand both the genetic drivers and the biological mechanisms behind response and adverse effect to anticoagulant medications.
- Pharmacogenomics of Inflammatory Bowel disease: We are investigating the genetic predictors of primary non-response to biologic therapies used in inflammatory bowel disease. Studies have implication for other autoimmune disorders that target the same pathways.
- eMERGE: We are involved in analyzing the GWAS and sequencing data specifically for genomics variation affect key pharmacogenomics gene in African Americans.
For lab information and more, see Dr. Perera's faculty profile and lab website.
Publications
See Dr. Perera's publications on PubMed.
Contact
Contact Dr. Perera at 312-503-6188 or the lab at 312-503-4119.
Ziarek LabExploring the protein structure/function/dynamics relationship, molecular mechanisms of allostery, and the rational design of molecular therapeutics and tools – with a long-standing interest in G protein-coupled receptors (GPCRs)
Exploring the protein structure/function/dynamics relationship, molecular mechanisms of allostery, and the rational design of molecular therapeutics and tools – with a long-standing interest in G protein-coupled receptors (GPCRs)
Research Description
G protein-coupled receptors (GPCRs) dominate the therapeutic market – being the targets for more than 30 percent of FDA-approved drugs. They also recognize most drugs of abuse including opioids, cannabinoids, LSD, cocaine, and methamphetamine. Unlike many signaling proteins that function as binary switches between 'on and off' states, GPCRs feature a ligand-independent basal activity that is increased or decreased upon orthosteric ligand binding, and then further regulated by allosteric modulators. A single receptor may specifically recognize several ligands and respond uniquely to each, creating a complex conformational landscape. There is immense therapeutic potential in the ability to tune receptor signaling using partial agonists, biased agonists or allosteric modulators – and it remains poorly understood and virtually untapped.
The long-term vision of the Ziarek Lab is to decipher the molecular mechanisms of GPCR allostery for rational drug discovery. The lab's current understanding of biased agonism derives primarily from cell-based functional assays that are easily complicated by receptor expression levels, off-target effects and cell type. Integrating biophysical studies of purified protein with this cellular information would permit a complete molecular understanding. Some research areas the Ziarek lab is currently making progress on are:
- investigating the conformational space of wildtype GPCRs using a combined biophysical and computational approach;
- characterizing the allosteric mechanisms of arrestin activation;
- developing novel 19F-NMR labels, pulse programs and NMR data analysis pipelines for expanding the molecular weight limits of NMR;
- testing if arrestin’s local disorder and conformational entropy play a role in receptor and scaffolding function; and
- actualizing the full potential of NMR to explore GPCR conformational dynamics using receptors compatible with E. coli expression systems.
For more information, see Dr. Ziarek's faculty profile and laboratory website.
Bumbak, F., Bower, J.B., Zemmer, S.C., Pons, M., Inoue, A., Paniagua, J.C., Yan, F., Ford, J., Wu, H., Robson, S.A., Bathgate, R.A.D., Scott, D.J., Gooley, P.R. & Ziarek, J.J. 2023. Stabilization of pre-existing neurotensin receptor conformational states by arrestin-1 and the biased allosteric modulator ML314. Nature Communications, 14(1):3328. 10.1038/s41467-023-38894-8.
- We used 13CεH3-methionine NMR spectroscopy to show that binding of phosphatidylinositol-4,5-bisphosphate (PIP2) to the receptor’s intracellular surface allosterically tunes the timescale of motions at the orthosteric pocket and conserved activation motifs – without dramatically altering the structural ensemble. A β-arrestin biased allosteric modulator transforms the NTS1:G protein complex into a concatenation of substates, without triggering transducer dissociation, suggesting that it may function by stabilizing signaling incompetent G protein conformations such as the non-canonical state. Together, this work demonstrates the importance of kinetic information to a complete picture of the GPCR activation landscape.
Bumbak, F., Pons, M., Inoue, A., Paniagua, J.C., Yan, F., Wu, H., Robson, S.A., Bathgate, R.A.D., Scott, D.J., Gooley, P.R. & Ziarek, J.J. 2023. Ligands tune the local and global motions of neurotensin receptor 1 (NTS1). Cell Reports, 42(1): 112015. https://doi.org/10.1016/j.celrep.2023.112015.
- Here, we expanded a novel method comparing experimental NMR data to density functional theory (DFT) quantum calculations. Our results suggest a role for sub-microsecond dynamics and conformational entropy in GPCR ligand discrimination. This approach sidesteps the isotopic labelling limitations imposed by eukaryotic expression systems. Future studies aim to test the generalizability of our results to the GPCR superfamily and quantitate the underlying motions.
- In this work, we introduce cysteine-reactive 19F probes at the intracellular tip of NTS1 transmembrane helix 6 (TM6). We observe a linear three-site exchange process that is both thermodynamically and kinetically remodeled by orthosteric ligands, arrestin, and a G protein mimetic. But whereas arrestin selects for pre-existing active-like substates, the G protein mimetic induces two new substates. Together, our study suggests i) a thus far unrecognized kinetic component to transducer selection, and ii) the available static structures do not represent the entire conformational ensemble observed in solution.
Publications
See Dr. Ziarek's publications on NCBI.
Contact
Contact Dr. Ziarek.
The Ziarek Lab is hiring highly motivated postdocs, graduate students and technicians.